The Lord has determined the laws of nature and the kinds of particles that can exist so that our universe has the right properties for life. Biology is possible because of chemistry, which is possible because of physics: the elementary particles God created and the rules governing them. A slight change in the properties of particles or the laws they obey, and atoms could not exist and biological life would be impossible. The intelligence needed to construct the right particles and laws such that biological life can exist is far beyond the combined mental capacity of all people who have ever lived. Quantum particles give us a bit of insight into the infinite mind of the Lord.
A Brief Review
We have been exploring elementary particles: those particles that are not made of any smaller particles. And we have examined two families of elementary particles: leptons and quarks. There are six varieties (called flavors) in each of these two families, and there are six corresponding anti-particles (six antileptons and six antiquarks). Our examination of leptons was straightforward because leptons can be studied in isolation. Furthermore, most people are already aware of the electron – the least massive charged lepton. The muon and tau are heavier versions of the electron, and then there are the neutral versions called neutrinos which have the ghostly ability to pass through ordinary matter.
Quarks are more complicated because they have “color charge” and respond to the strong force. This means that quarks are never found in isolation. They always combine into color-neutral composite particles called hadrons. Quarks have fractional electric charge (+2/3 for the up, charm, and top quark, and -1/3 for the down, strange, and bottom quark) and will only group in such a way as to make a hadron with integer charge.


Each quark comes in one of three color charges: red, green, or blue. These have nothing to do with our visual experience of color, but merely refer to three types of charge. Antiquarks come in one of three color charges: anti-red, anti-green, and anti-blue. Antiquarks have opposite electric charge from quarks, coming in either -2/3 or +1/3 varieties. There are two ways in which quarks and/or antiquarks can combine to form a color-neutral hadron; and hence there are two types of hadrons: baryons and mesons. We covered baryons in the previous article; a baryon is always made of exactly three quarks (red, green, and blue). There are also antibaryons made of three antiquarks (anti-red, anti-green, and anti-blue). Mesons are made of one quark and one anti-quark of opposite color (such as red and anti-red). Before we examine the properties of mesons, we need a bit more information on the strong force.

The Strong Force Revisited
The nuclear strong force is what is responsible for holding together the three quarks in any baryon such as the proton. It’s much like the electric force, but is stronger and involves six charges rather than just two. So, we need to understand how these six color charges interact. As with the electric force, like charges repel and opposite charges attract. But what about non-opposite colors? How do they relate?
First, the strength of the force is twice as strong for same color or anti-color as it is for “other” colors. In other words, the force between a red particle and another red or anti-red particle will be twice as strong as the force between a red and a green or anti-blue particle. So, then we just need to know if the force is attractive or repulsive. The pattern is as follows: (1) each color is strongly repelled by the same color (2) weakly attracted to the other two colors, (3) strongly attracted to its own anti-color, (4) weakly repelled by the other two anti-colors.
For example, a red particle will attract a blue or green particle and will doubly attract an anti-red particle. A red particle will repel an anti-green or anti-blue particle, and will doubly repel another red particle. Thus, the force between the red, green, and blue quarks in any baryon is attractive, but has only half the strength of the force between a red particle and an anti-red particle.
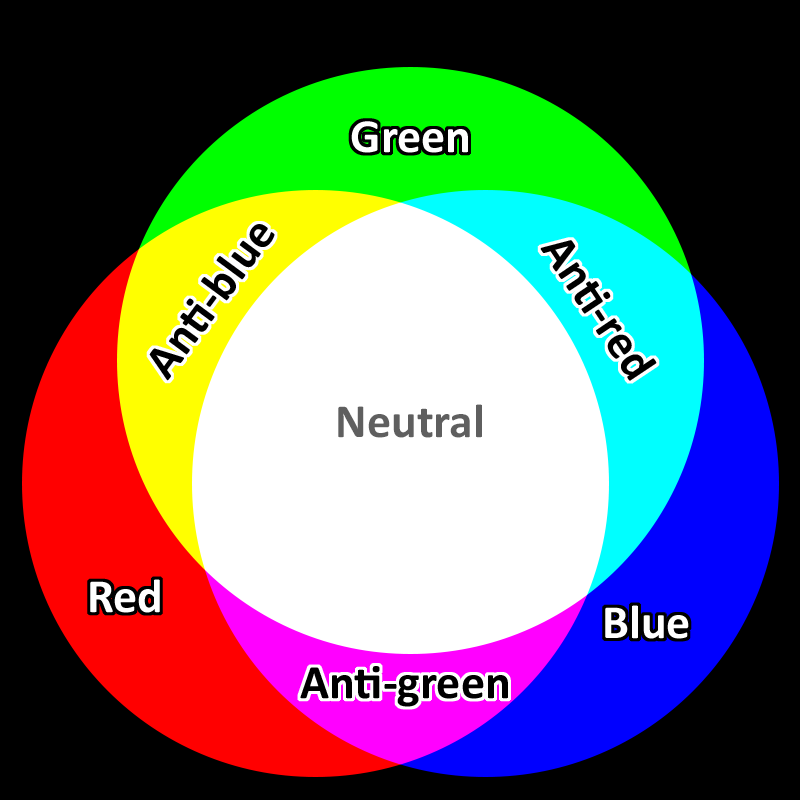
Another way that a quark can combine to form a composite particle is to join with one antiquark. When a quark and antiquark combine, the composite particle is called a meson. Recall that quarks and antiquarks will only combine in such a way as to make a color-neutral particle. Therefore, the quark and anti-quark in any meson will always have opposite color charges: red and anti-red, green and anti-green, or blue and anti-blue. In fact, a single meson can oscillate between these color combinations. The resulting meson is therefore color-neutral. In fact, we can now see that if the antiquark did not have the opposite color charge of the quark, the two particles would repel and would not form a meson. That is, a red quark will repel an anti-blue or anti-green antiquark.

All mesons have an integer electric charge. Adding the charge of any one quark to any one antiquark will always result in a total charge of -1, 0, or +1. And indeed, all mesons have one of these three electric charges.
Pions
The first meson was discovered in 1947, and is called a pi-meson or pion.[1] There are actually three particles in the pion family: a positive pion, a neutral pion, and a negative pion. Let’s first consider the positive pion (left side on the chart below). It is comprised of one up quark, and one down antiquark with opposite spin alignment (and opposite color charge). Adding the charges of the constituent quark/antiquark pair, we find the total charge is +1: the same as the proton.

At first, we might suspect that the pion would have about 2/3 the mass of the proton since it is made of two particles (one quark and one antiquark) whereas the proton has three quarks. But recall, the strong force is twice as strong between opposite colors as it is between “off” colors. Thus, the binding energy between a red quark and anti-red antiquark in a pion is much stronger than the binding energy between the three colored quarks in a proton. This binding energy is negative, which further reduces the mass of the system. This is the “mass defect” we discussed in the previous article. Consequently, mesons tend to be lighter than baryons with similar quark flavors because the stronger binding energy produces a larger mass defect.
The negative pion consists of one down quark and one up antiquark (right side in the above illustration). These combine to form a pion with a charge of -1: the same as the electron. The positive and negative pions have exactly the same mass, and are anti-particles of each other. Since positive and negative pions are equally abundant in the universe, it is meaningless to ask which one of them is “matter” and which is “antimatter.” Each is the anti-particle of the other. There are no anti-mesons, because each meson is one quark and one antiquark. So, the anti-particle of any meson is just another meson (and sometimes the same meson).[2]
There is also a neutral pion. An up quark and an up antiquark would combine to make a zero-charged pion. But so would a down quark and a down antiquark. So, which is the combination for the neutral pion? The answer is both. Until it is observed, the wavefunction of the neutral pion acts like a combination of both states. When observed, there is a 50% chance a neutral pion will be an up quark and an up antiquark, and a 50% chance it will be a down quark and a down antiquark. The slight mass difference between the up and down quarks/antiquarks is easily absorbed in the binding energy. So, the neutral pion has an indeterminate composition unless and until it is observed. Several other neutral mesons also have an indeterminate composition: these include the rho (ρ), the eta (η), the K (S and L versions), and the omega (ω) mesons. Each of these mesons is its own antiparticle.
Mesons are Bosons
All the particle families we have studied previously (leptons, quarks, and baryons) are fermions: particles with half-integer spin. Fermions obey the Pauli exclusion principle: they cannot exist in the same quantum state (same place, same time, same spin, etc.). Baryons are fermions because the sum of three ½-spin particles always results in a particle with either ½ or 3/2 spin.
However, a meson has only two particles. And since each will either be spin-up (+1/2) or spin down (-1/2), a meson will always have a total spin of either 0 or 1 (in the ground state). A meson is spin-0 if the quark and antiquark have opposite spin states; a meson is spin-1 if the quark and antiquark have the same spin state.
If the quark and/or antiquark have orbital motion, this will create higher spin states in integer steps (2, 3, 4, …). But the result is always an integer. So, all mesons always have an integer spin, and are therefore bosons. Recall that bosons are not constrained by the Pauli exclusion principle; multiple bosons can exist in the same quantum state. This is rather fascinating because every meson is comprised of two fermions. And fermions must obey the Pauli exclusion principle. It seems strange that mesons can (and do) violate the Pauli exclusion principle even though they are made of particles that cannot.
As with baryons, the total spin and mass of a meson is affected not only by their quark/antiquark composition, but also by whether those quarks have aligned or anti-aligned spin states. The rho meson has exactly the same quark/antiquark composition as the pion. But with the rho, the spin states are aligned, resulting in a meson of spin one, whereas the pions have a spin of zero. Aligned spin states have higher (positive) energy, and thus the rho mesons are considerably heavier than the pions despite their identical quark composition.

The pion and rho meson are among the lightest mesons because they contain only the lightest two flavors of quarks/antiquarks: the up and down. The next least massive flavor is the strange quark/antiquark. K-mesons (kaons) consist of one up or down quark or antiquark, and one strange quark or anti-quark. Kaons can be spin zero (if their constituent quark and antiquark have opposite spin) or spin one (if the quark and antiquark have aligned spin states). The spin-0 versions of the neutral kaon can exist in an indeterminate composition (much like the neutral pion), but there are two possible wavefunctions and these affect the lifetime of the particle. These two versions are called the K-short and K-long.
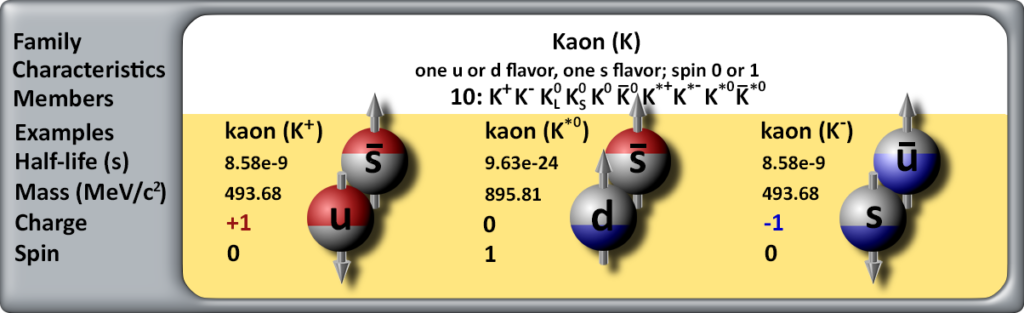
There are ten families of mesons. Each meson family is named after a lowercase Greek letter or an uppercase English letter (or both as is uniquely the case for the J/psi meson). These are: the pion (π), rho (ρ), kaon (K), eta (η), D, B, omega (ω), phi (φ), J/psi (J/ψ), and the upsilon (υ). Some families contain only one member. For example, the J/psi meson consists of a charm quark and charm antiquark with aligned spin. Likewise, the phi meson is a spin-1 meson consisting of a strange quark and strange antiquark.
Meson Decay
Mesons can decay – they can spontaneously change into other combinations of particles. The kinds of particles mesons can decay into is determined by the conservation laws. Recall that a particle can only decay into a less massive particle, and the combined charge and spin states of the daughter products must match the charge and spin state of the original. Furthermore, lepton number and baryon number are conserved. Mesons have a lepton number of zero, and a baryon number of zero. Thus, if they produce a lepton in a decay, they must also produce an anti-lepton so that the total lepton number remains zero.
The reason the lightest charged lepton (the electron) cannot decay is because it would violate lepton number. Likewise, the lightest baryon (the proton) cannot (in isolation) decay because the only things lighter are non-baryons, and that would violate baryon number. We might at first suspect that there is a similar rule for mesons. However, there is no “meson number,” or at least it is not a conserved quantity. Thus, mesons can decay into non-mesons. Even the lightest meson, the neutral pion, can decay.
Therefore, there are no stable mesons. All mesons have a fleeting existence. In fact, the longest-lived meson, the K-Long, has a mean lifetime of only 51 nanoseconds – that’s 51 billionths of a second. The second longest-lived mesons are the charged pions (either positive or negative), each of which has a mean lifetime of only 26 nanoseconds.
The positive pion often decays into an anti-muon and a muon neutrino with opposite spin state from the anti-muon. We can confirm that this obeys all the conservation rules: the anti-muon and muon neutrino are less than the mass of the pion (with the kinetic energy carrying away the difference), the total charge before and after is +1, the total spin state before and after is zero, and the total lepton number before and after is zero. We can see this is an allowed decay, and therefore it can occur.
How might a neutral pion decay? It is the lightest meson, so it cannot decay into another meson. Recall this particle can be either an up quark and up antiquark, or a down quark and a down antiquark. The total charge and total spin are each zero. The lepton and baryon numbers are zero. So, this must be true of the sum of the daughter products as well. One option would be to decay into two photons with opposite spin states. That obeys all the conservation laws, and in fact is the most common decay of the neutral pion. Another possibility is to decay into one photon (spin state +1), an electron and positron (each spin state -1/2). The total mass is less than the pion, and the total charge and spin state are each zero. That decay is the second most common.
Decay in Other Particles
Now that we understand mesons and their properties, we can read and understand charts depicting the decay of essentially all other particles. In the article on leptons, we mentioned one possible decay of the tau (the most massive lepton) into other leptons. But the most common decay pathways of the tau involve mesons. The tau most commonly (25.5% of the time) decays into a charged pion, a neutral pion, and a tau neutrino. These pions then decay as discussed above.
Likewise, the decay of baryons (although always resulting in at least one other baryon) often involves mesons. Consider the delta baryons. The neutral delta has the same quark composition as the neutron, but has greater mass and a spin of 3/2 due to the fact that all three of its quarks are spin-aligned. The neutral delta commonly decays in one of two ways. First, it can decay into a neutral pion and a neutron. Alternatively, it can decay into a negative pion and a proton. The daughter products have less mass than the original, and you can confirm that the total charge is conserved, as is baryon number. The resulting pion will have orbital angular momentum relative to the resulting nucleon, resulting in a spin of 1, even though the pion itself has an intrinsic spin of zero. So, total spin is also conserved.
Exotic Hadrons
In addition to pure baryons and mesons, there are other possible quark combinations that result in a color-neutral composite particle. The pentaquark is a collection of four quarks and one antiquark. As such, it is essentially a combination of one baryon and one meson. Whether this exists as a “molecule” of a baryon and meson, or whether all five particles equally interact is currently debated. Several varieties of pentaquarks have now been created in particle-collision experiments. Naturally-occurring pentaquarks have not been discovered, but may be formed in high-energy cosmic events such as a supernova.
The tetraquark is a combination of two quarks and two antiquarks. We can think of it as essentially the combination of two mesons. Several varieties of tetraquarks have been detected in particle-collision experiments over the last several years. Like the pentaquark, the tetraquark has not been observed to form under natural conditions. Both pentaquarks and tetraquarks are unstable, decaying into other particles almost immediately after they are formed.
Physicists have also proposed the possible existence of a quark nugget. This hypothetical particle consists of large numbers of the three lightest flavors of quarks (or antiquarks): the up, down, and strange, in roughly equal proportions. The resulting particle would be extremely massive, and quite possibly stable. Some scientists have suggested that quark nuggets may be responsible for dark matter – an unseen substance known only by its gravitational effects on visible matter. Currently, quark nuggets have not been definitively detected.
Mesons and the Residual Strong Force
Although mesons have only a fleeting existence, they are essential in order to have atoms heavier than hydrogen. Recall that the quarks within a proton or neutron are held together by the strong force. But this force has extremely limited range. Beyond the radius of the proton or neutron, there is only a residue of color charge due to imperfect cancellation of the three colors due to the different positions of the three quarks. This residual color field has energy. And from that energy, mesons can be created. Since pions are the least massive mesons, they are the most likely to form in this field. However, rho mesons can also contribute.
These mesons can pass from one proton or neutron to a neighboring proton or neutron where they are absorbed. This process results in an attractive force between nucleons. Essentially, a cloud of constantly forming and unforming mesons surrounds a proton or neutron, and this has the effect of extending the strong force enough so that protons and neutrons can stick together in the nucleus of an atom.
If a neutral pion forms from the energy field and passes from a proton to a neutron, it produces an attractive force between both particles, but leaves them unchanged. On the other hand, a charged pion can swap the proton and neutron. Suppose a positive pion is emitted from the proton; to conserve charge it would have to convert an up quark to a down quark in the proton, to obtain the pion’s +1 charge. This converts the proton to a neutron. This pion is then almost immediately absorbed by a neighboring neutron, changing one of its down quarks to an up quark in the process. The pion ceases to exist when absorbed, but the neutron has become a proton. Notice that this process cannot change the number of protons and neutrons in a nucleus; it can only swap their positions.
Thus, mesons are constantly being emitted and immediately absorbed by the protons and neutrons in the nucleus. This has the effect of extending the strong force just a bit – enough to keep the nucleus together. Without mesons, the only atoms that could exist would be hydrogen.
So, mesons are just another link in the chain of quantum particles that the Lord created in order for physics to be what it is. And the Lord causes physics to be what it is so that chemistry will be what He decrees. And chemistry needed to be just right in order for biological life forms to exist. Our minds are not capable of considering the infinite number of possibilities for particles, the rules governing them, and the potential chemistry that results. But God’s mind is and has. In the next (final) article on quantum particles, we will look at elementary bosons and their essential role as the glue by which God holds universe together.
[1] Recall that all baryons (except nucleons) are also named after Greek letters, and when written these letters are always capital. Each meson family is indicated either by a lower-case Greek letter or a capital English letter. The J/psi meson uses both.
[2] Contrast this with baryons. Baryons differ from antibaryons by composition. Baryons are made of three quarks, whereas antibaryons are made of three antiquarks. Since a meson is a quark/antiquark pair, reversing the charges results in a meson.