In the core of every atom is a nucleus consisting of one or more protons, and (except for hydrogen) some neutrons as well. Protons and neutrons are responsible for most of the mass of an atom. The electrons “orbit” around this central nucleus, generally in equal numbers to the protons, which makes the overall atom electrically neutral. Electrons are elementary – meaning they are not made of any smaller particles. But protons and neutrons are composite particles; they are made of smaller particles called quarks.
Quarks vs. Leptons
Since God is a God of order, the various objects He created can be classified in natural families on the basis of similarities and differences. In the previous article we examined the family of particles called leptons which are very low-mass particles (except for the tau). We found that there are exactly six types (called flavors) of leptons, three of which possess an electrical charge of -1 (the electron, muon, and tau), and three of which are uncharged (the neutrinos). There are three generations of leptons, with two particles (differing by electric charge) in each generation.
The particles that comprise protons and neutrons are called quarks. And, as with the leptons, there are exactly six types (flavors) of quarks. Furthermore, there are three generations, with two quarks in each generation (and which differ by electric charge). Just as each lepton has a spin of ½, likewise each quark has a spin of ½. So, quarks and leptons are both fermions – non-integer spin particles that obey the Pauli exclusion principle. Like leptons, quarks have no detectable size. In all these ways, the similarity between quarks and leptons is remarkable.
But there are some differences too. And these differences make quarks a bit more complicated than leptons. We might think of leptons as the introverts of the universe. They are perfectly happy by themselves and can found apart from any other particles. Of course, the charged leptons can interact with other particles; electrons can orbit around the nucleus of an atom, but they tend to keep their distance. And of course, the neutrinos are just downright anti-social. They pretty much ignore all other particles and each other unless there is a direct collision.
Quarks, on the other hand, are like extroverts. They are always surrounded by and interacting with other particles. As far as we know, quarks are never found in isolation. They only exist in combination with other particles. We will see the reason for this later. For now, it is sufficient to know that quarks always occur in combinations. Often, they will occur in groups of three to form composite particles like protons and neutrons. The name “quark” refers to this three-ness. It is taken from the first line of a poem about King Mark written by James Joyce included in Finnegan’s Wake: “Three quarks for Muster Mark!”
Properties of Quarks

From the table, we see that quarks fall into three generations with two particles in each, for a total of six flavors, just like leptons. But there are two remarkable differences. First, the quarks are much more massive than the leptons.[1] Furthermore, notice that the chart lists two different masses for each quark. This is because quarks have a strong energy field surrounding them; that energy has mass. The “constituent mass” is the total mass of the quark along with its field. The “current mass” is the mass of the actual quark if we could somehow separate it from its energy field – which we really cannot do. So, the constituent mass is the relevant mass in practical applications, and the only one we will use going forward.
Second, quarks have fractional electric charge. For example, the down quark is negatively charged, but has only 1/3 the charge of an electron. The up quark has a positive charge, but it is 2/3 the charge of a proton. We have been using color to illustrate electrical charge with blue denoting negative charge and red denoting positive charge. So, the fractional charge of quarks is shown as a sphere only 1/3 or 2/3 full of charge.[2]
There are six types of antiquarks as well. They are identical to quarks in every way except their charges are reversed.[3] So, the up antiquark has a charge of -2/3 while the down antiquark has a charge of +1/3. As far as we know, quarks and antiquarks are the only particles with a non-integer charge.
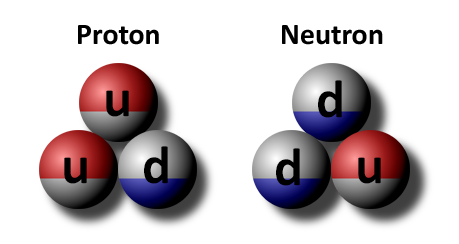
Although quarks themselves have fractional charge, they will only combine in ways to make a composite particle with an integer charge. They often cluster in groups of three. For example, the proton is made of two up quarks and one down quark. Adding 2/3 plus 2/3 plus -1/3 adds up to +1. So the proton has a charge of +1. The neutron is made of one up quark and two down quarks for a total charge of (2/3 – 1/3 – 1/3) = 0. By the way, the “up” and “down” are simply names and have nothing to do with spin state.[4]
As with the charged leptons, the higher generations of quarks are progressively more massive, with the top quark weighing in at 189 times the mass of a proton! Recall from the previous article that the more massive particles tend to be unstable. They will spontaneously decay into less massive particles as long as they can obey all the conservation laws. This is true of quarks as well. Sure enough, the top and bottom quarks have the fastest decays, followed by the charm and strange quarks. The up and down quarks are potentially stable, but their stability depends on how they are grouped. Remember, quarks are never alone. Hence, their proximity to other particles can affect whether or not they decay.
Color Charge and the Strong Force
The nucleus of a helium atom contains two protons and two neutrons bound together. But what is binding them? We understand that electrons tend to stay with their atom because they are negatively-charged and are therefore attracted to the positively-charged nucleus. But the two protons are each positively charged. So, shouldn’t they repel each other? Physicists recognized this problem very early and reasoned that there must be some other force between the protons – an attractive force – that is even more powerful and therefore overcomes their electric repulsion. This force is also responsible for holding together the quarks within a proton. It is called the strong nuclear force, or simply the strong force.
The strong force is similar to the electric force in many respects. Both forces can cause two particles to attract or repel. But there are some differences. First, the strong force is much, much stronger than the electric force (hence the name). Second, the strong force has an extremely limited range.[5] In fact, it really doesn’t extend much beyond the nucleus of an atom. This is why we don’t observe the strong force in our observations of macroscopic objects.
Third, the electric force contains only two types of charge, which we call “positive” and “negative.” But the strong force has six types of charge. Physicists use color to represent these charges. Three of these colors are red, green, and blue. The other three are called anti-red, anti-green, and anti-blue. (We often depict these respectively as cyan, purple, and yellow – the opposite or complimentary colors of red, green, and blue). These color charges have absolutely nothing to do with what we normally think of as “color” in terms of our visual experience. The red, green, and blue terms are simply labels we use to describe three of the six types of charge associated with the strong force. But we will see later that there is a connection to visual color when it comes to the way these color charges add. So, color is a useful analogy.
In a later article, we will explore how these six charges work in terms of attracting or repelling the others. Here, we will only focus on the first three: red, green, and blue. As with the electric force, like charges repel. So, a particle with red color charge would repel another particle with red color charge. But different colors attract. So, a particle with red charge will attract a particle with blue or green charge. Likewise, blue will attract red or green; green will attract red or blue.
Each of the six types of quarks comes with one of these three color charges. So, in a way, there are actually 18 quarks since each of the six flavors comes with red, green, or blue color charge. Conversely, each antiquark comes in one of the three anti-colors: anti-red, anti-green, or anti-blue. But the particles we will discuss in this article and in the next are made only of quarks, not anti-quarks. Thus, we need only address three of the six color charges – red, green, and blue.
What happens when you take a red spotlight, a green spotlight, and a blue spotlight, and shine them all at the same spot? You get white. Likewise, a particle that is made of an equal amount of red, green, and blue color-charged particles will have an overall color of “white” – it will be color-neutral. This is exactly the case with the proton and neutron. The proton has one red quark, one green quark, and one blue quark. Since these three charges are mutually attractive, the three quarks stick together making a color-neutral proton. Likewise, each neutron is made of one red quark, one green quark, and one blue quark.
In fact, the quarks within a proton are constantly swapping color charges with each other. We’ll see how that works in a later article. But at any given moment, one of the quarks has red color charge, another has green, and the remaining quark is blue. Composite particles are always color-neutral. This seems to be a law of nature.
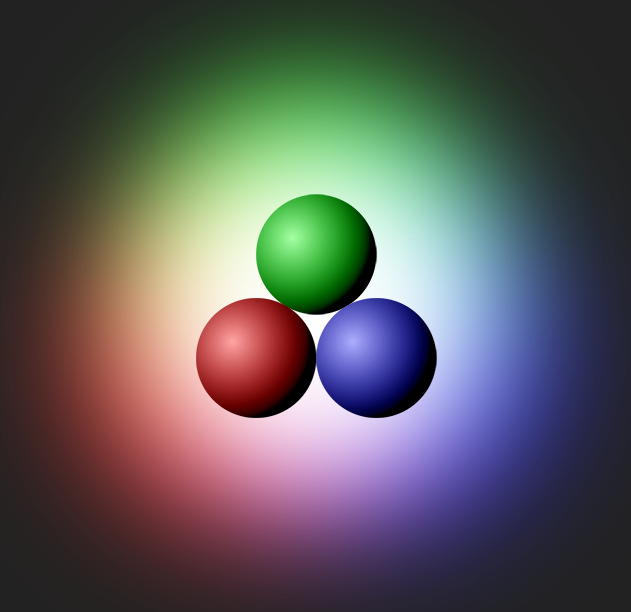
If each of the two protons in the nucleus of a helium atom is color-neutral, then why do they attract? Well, in a way, they don’t – at least not directly in the same way that quarks within a proton attract. Although the three quarks add together to form a color-neutral proton, the cancellation isn’t perfect because these three quarks have slightly different positions. This results in a residual field of color charge extending just beyond proton. This is sometimes called the residual strong force. The distance between the two protons in helium is just within the range limit of the residual strong force.
The reason that leptons do not have any of these complications is because they are all color-neutral. They possess no color charge, and therefore leptons do not experience the strong force. Nor do photons. But quarks possess color charge, and since the strong force is far more powerful than any other force, this explains why quarks come in groups of three.[6] In fact, the amount of energy required to separate a quark from the other two is more than sufficient to create new quarks/antiquarks. So, a new quark will form and instantly fill the vacancy. Therefore, quarks cannot be isolated. They always come in groups.
For this reason, we really need to examine the composite particles that quarks (and/or antiquarks) tend to form. These composite particles are called hadrons. The flavors of quarks or antiquarks in a hadron, along with the way in which the quarks combine in terms of their spin states, will determine the properties of the hadron. Since composite particles must be color-neutral, there are two ways in which quarks or antiquarks will combine, and therefore two types of hadrons. First, quarks can combine in groups of three (one red quark, one green quark, and one blue quark) into a particle we call a baryon. Another way to generate a colorless hadron is to combine one quark with one antiquark of opposite color charge (such as a red quark and an anti-red antiquark) into a particle we call a meson. In the next article we will examine the properties of baryons. Baryons are the substance of the cosmos.
[1] Except of course for the tau. All quarks (by constituent mass) are heavier than all leptons excluding the tau. But even the bottom and top quarks are heavier than the tau.
[2] This is simply a visual way to remember their charges. Quarks are thought to be points of no size, not spheres. And, of course, they have no literal color in the sense of reflecting various light frequencies.
[3] This charge reversal includes “color charge” as discussed later.
[4] That is, the up quark can be either spin-up (+1/2) or spin-down (-1/2). And likewise with the down quark.
[5] In contrast, the range of the electric force is (in principle) infinite, although it diminishes in strength as the square of the distance.
[6] Alternatively, quarks can come in quark/antiquark pairs. These are mesons – to be covered in a later article. Mesons are also color-neutral.