“And God made the two great lights, the greater light to govern the day, and the lesser light to govern the night; He made the stars also.” (Genesis 1:16).
The purpose of the Sun, the reason it was created, was to govern the day by setting its boundaries, to give light upon the Earth, and to separate light from darkness (Genesis 1:14-19). It is for our benefit! The sun is not a god, although many ancient cultures worshipped it as such. It is not the primary or ultimate source of life. Rather it is a creation of the God who is the primary, ultimate source of life. Perhaps this is why God Himself provided light on Earth for the first three days, delaying the creation of the sun until day 4. It shows that the sun is not primary. Furthermore, God does not even call the sun and moon by their names in Genesis 1. Perhaps this is to reduce our temptation to worship these objects as gods. They are not persons, but mere objects to be named later (Psalm 136:8-9).
The sun is a creation of God to give light upon the Earth and to govern the day and separate light from dark. And the sun does exactly these things. It illuminates the Earth and governs the day by determining its boundary; if the sun is above the horizon, it is day, otherwise it is night. But the way in which the sun fulfills its God-ordained role is fascinating, and altogether unexpected.
We might at first suspect that the sun is relatively small, close to the Earth, and circles the Earth every 24 hours. After all, that’s all it would take to accomplish its purpose. A human engineer would not waste resources by building something that is far in excess of its intended purpose. And indeed, many ancient cultures believed that the sun was an object much smaller than Earth and that it circled the Earth once every day.
But God is not an engineer; He is God. His thinking is infinitely superior to ours. And since He creates out of nothing, He is not limited by resources. So, we should not suppose that God must do things the way we would. That would be bad theology and bad science. And astronomy is full of counter-examples. The sun does all the things required of it, but it does far more than this. Not only does it illuminate and determine daytime for Earth, but for all the planets and moons of the solar system. Furthermore, this greater light is far larger than the Earth – over 100 times the diameter of Earth. And it is not the sun that goes around the Earth, but the Earth that orbits the sun. The Lord certainly has a wonderful sense of irony. And He has ways of humbling us when we feel that the entire universe should revolve around us. It doesn’t!
Early Measurements
The discovery that the sun is much larger than Earth goes all the way back to Aristarchus, a Greek mathematician and scientist who lived in the third century B.C. Aristarchus used geometry to compute the distance to and size of the moon for the first time. The moon is about a quarter of the Earth’s diameter, and orbits at a distance of 240,000 miles. Aristarchus then used this method to compute the distance to the sun. Although the precision of his calculation was low due to the crudeness of astronomical observations of the time, Aristarchus rightly concluded that the sun was significantly larger than the Earth. He reasoned that it would be absurd for the large object to circle around the small one. So, he was the first person in recorded history to propose that the Earth orbits the sun. And of course, he was right.
Interestingly, his idea of a heliocentric (sun-centered) solar system was not largely accepted at the time. Centuries of assumptions that Earth is the stationary center of the universe are not easily abandoned. And frankly, human intuition often fails us where physics is concerned. After all, if we are really on a sphere hurtling around the sun at 67,000 miles per hour, wouldn’t we feel something? Why is there no wind? Why do we not see nearby stars appear to shift relative to distant stars as we orbit the sun (an effect called parallax)?
These were some of the objections proposed at the time. And they are easily answered. We don’t feel motion because human beings do not have the capacity to sense velocity, only changes in velocity (such as vibration, acceleration, and rotation). There is no 67,000 mile-per-hour wind because the air moves with the Earth; space is a vacuum. We don’t readily see parallax because the stars are far more distant than ancient people could have ever imagined. But today, we can indeed detect parallax with telescopes. Interestingly, Aristarchus himself correctly supposed the answers to all the objections to heliocentrism. But since there was no way to test his proposals, heliocentrism remained simply an untested and unpopular hypothesis.
In the 1500s, Copernicus revived the idea of the heliocentric solar system. Over the next centuries, observations proved the Earth-centered (geocentric) model to be wrong, and heliocentrism was confirmed. The invention of the telescope also dramatically improved our knowledge of astronomy. Galileo not only looked at the planets, moon, and stars with his telescope, but the sun as well! Of course, today we know this is very dangerous. Looking directly at the sun with or without a telescope can cause blindness because the sun emits ultraviolet rays that damage the retina of the eye. But no one knew about the existence (to say nothing of the danger) of ultraviolet light at the time of Galileo. Today, we have special filters that allow us to view the sun safely. And closeup images of the solar surface reveal lots of interesting features, such as sunspots and granulation.
Spectroscopy
Much of what we know about the sun is due to a technological invention of the early 1800s: the spectroscope. This instrument, invented by Joseph von Fraunhofer, splits white light into its various constituent wavelengths, which we perceive as color. A prism does this too, but the spectroscope does this far more effectively, by spreading the colors much farther apart. Fraunhofer used this device to analyze the light coming from the sun, and made a remarkable discovery. He found that certain very specific wavelengths/colors are missing or greatly suppressed from the sun’s light. Hence, the spectrum (the “rainbow”) produced by the sun is contaminated by a number of dark lines. We don’t see these dark lines in an ordinary rainbow in the sky because the colors are far too close together, and the lines are very thin. But a spectroscope reveals hundreds of thin lines corresponding to “missing” colors. Today, we refer to these as Fraunhofer lines. But what could they mean?
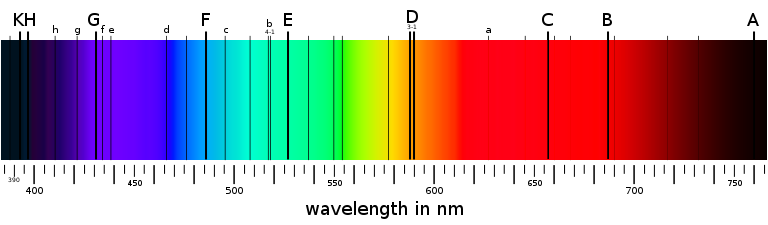
Fraunhofer also used his spectroscope to study light produced by fire, and found that its spectrum was quite different from the sun’s. He also examined the light from stars, and found dark lines similar to the sun, but not always in the same arrangement. Scientists later found that heating up a specific gas to a specific temperature would produce a very specific and consistent spectrum. Thus, the spectrum of an object tells us something about both the temperature and composition of its source! The locations of the lines in a spectrum are an atomic “fingerprint.” The dark lines found in the sun’s spectrum and those of other stars correspond to wavelengths of light that match atomic energy level transitions, which are determined by the element. Thanks to spectroscopy, we now know the composition of stars.
Some of the dark lines in the sun’s spectrum correspond to the element Hydrogen. Hydrogen gas is the main constituent of the sun, and of all stars. In 1868, astronomers detected spectral lines in the sun that did not correspond to anything as yet discovered on Earth. It was a new element, found only on the sun and apparently not on Earth. And since the Greek term for the sun “helios,” this “sun element” was named helium. Of course, later, helium was found on Earth and when heated, its spectrum matches the lines seen on the sun. But helium was indeed discovered on the sun before it was found on Earth. Helium is the second most abundant element in the sun, and in all stars.
We also know the temperature of the sun’s surface by spectroscopy. The strength of the spectral lines (their thickness and darkness) allows us to know the precise temperature of the solar surface. We know that the sun’s visible surface is around 6000 degrees Celsius. This is good, repeatable science because we can also heat up various gases to various temperatures in a laboratory, and observe the kind of spectrum that results.
Modern Solar Physics
Building on the hard work of those scientists who preceded us, we now know a great deal about the sun. It is an approximately spherical ball of hydrogen and helium gas, 865,000 miles in diameter, and held together by its own gravity. Some people are surprised to learn that gas has gravity, but in fact everything that has mass has gravity. The more mass, the greater the gravity. And the sun has a great deal of mass. In fact, the sun is about 333,000 times the mass of the Earth! The sun comprises over 99.8% the mass of our entire solar system.
To put this in perspective, imagine that a 10-pound bowling ball represents the mass of the sun. To scale, the mass of all the other objects in our solar system – all the planets, moons, asteroids, comets – would be equivalent to the combined mass of a penny and a nickel. The planet Jupiter would be the nickel.
The visible surface of the sun is called the photosphere. There are various features on the photosphere, the most well-known being sunspots. Sunspots actually emit light, but they appear dark because they give off much less light than the surrounding regions because they are colder. Sunspots have a temperature of around 4000 degrees Celsius, in contrast to the rest of the photosphere which is around 6000 degrees. Through detailed spectroscopy, we know that sunspots are associated with powerful magnetic fields. Magnetic fields can inhibit the movement of plasma – gas so hot that the electrons are not bound to atomic nuclei. But the movement of plasma is what provides heat for the photosphere, as hotter material from below is circulated to the surface. When a powerful magnetic field develops on a portion of the photosphere, the gas is prevented from circulated, which means it cannot get energy from below. So it cools off as it radiates energy into space. Hence, magnetic fields are the cause of sunspots. A sunspot is typically about the size of the Earth.
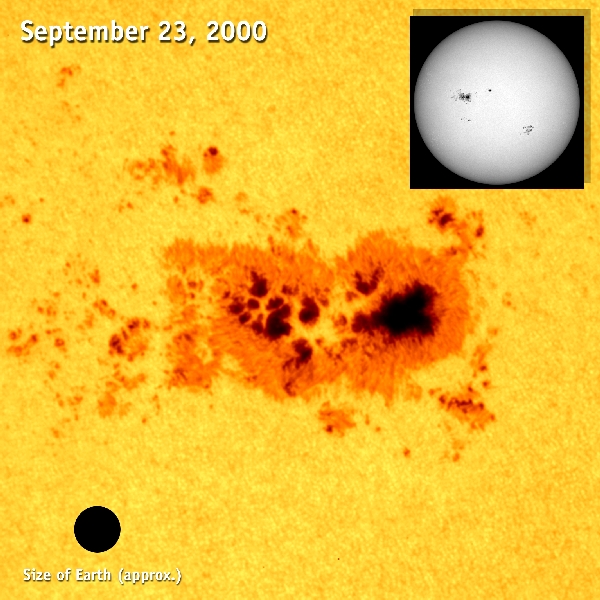
The sunspots undergo a 22-year cycle, consisting of two 11-year sub-cycles. Hence, every eleven years, the sun tends to have a lot of spots, followed by a period where it has very few spots. This is caused by a magnetic cycle. The sun has a dipole magnetic field, just like the Earth, with magnetic poles very near the rotation axis. But, the sun’s magnetic poles reverse every 11 years, which starts a new sunspot sub-cycle. Sunspots themselves tend to occur in magnetic pairs, but with an east-west orientation. So for example, most spots in the sun’s northern hemisphere will have a north magnetic spot east of a south magnetic spot, with the opposite in the southern hemisphere. Eleven years later, the polarity reverses, and spots in the northern hemisphere will have a south magnetic spot east of a north magnetic spot, with the opposite in the southern hemisphere. For a ball of gas, the sun is amazingly complicated!
The photosphere also has a small-scale mottling pattern called granulation. Appearing a bit like an irregular honeycomb, these are convection cells. They constantly bring up hotter material in their interior which circulates and drops back down at the perimeter of the cell. Each of these cells is about the size of Texas.
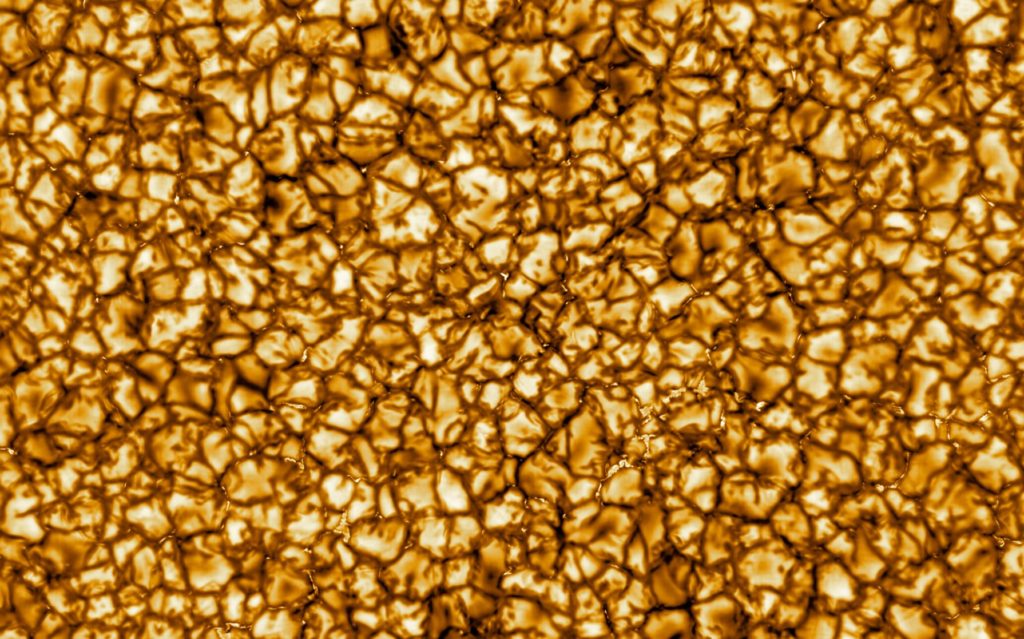
There are three other scales of convection on the sun, but they are harder to see directly. Supergranules are a much larger version of granules, but the circulation pattern is the same: upwelling material in the center and downward motion at the perimeter. Each supergranule is about the size of Earth. Intermediate between these two scales are mesogranules. A fourth, much larger type of convection unit is called a giant cell. These are about one third the radius of the sun. My graduate school research focused on detecting the boundaries of these giant cells using data from the SOHO spacecraft.
Solar Layers
The sun has layers, like an onion. There are two layers above the photosphere called the chromosphere and the corona. We cannot normally see these layers because they are much fainter than the photosphere. But they can be seen by eye during a total solar eclipse. When the moon completely covers the photosphere, we can suddenly see an extremely thin vividly red layer surrounding it; that’s the chromosphere. I had the privilege of seeing this during the 2017 eclipse, and I can’t believe how beautiful it was. Beyond the thin chromosphere is a much larger region called the corona. It can be many sun diameters in size, and is irregularly shaped. It looks almost like a bluish wind streaming away from the sun. The corona consists of extremely rarified hydrogen plasma that is up to 1 million degrees Celsius – much hotter than the photosphere below. We now have instruments that can view the chromosphere and corona directly without waiting for an eclipse.
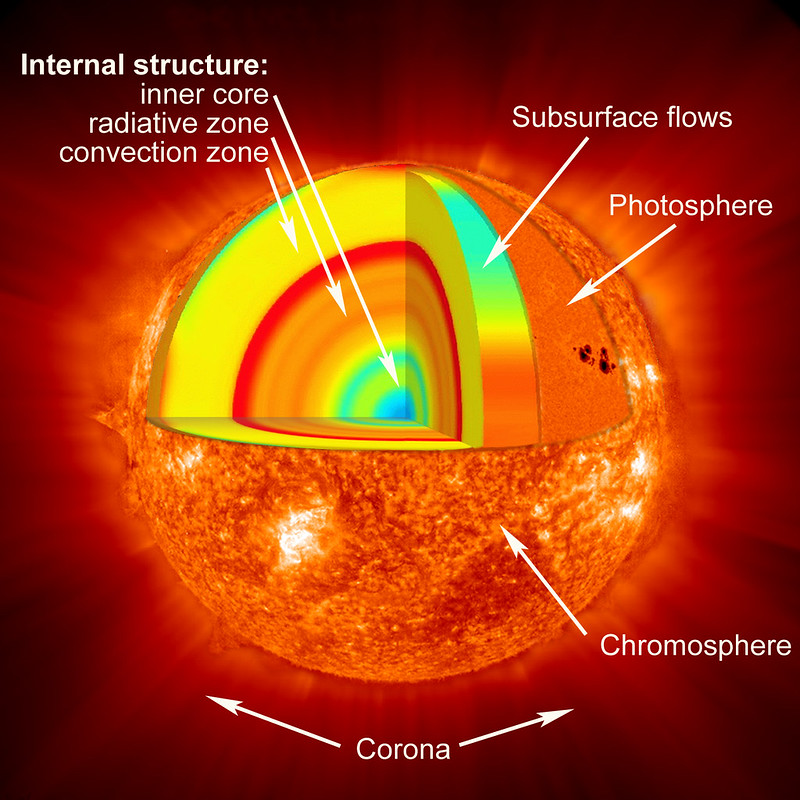
Amazingly, we also know something about the interior layers of the sun. The photosphere is actually the top of a layer called the convection zone, which occupies the outermost third of the sun. The convection zone is characterized by circulating cells of gas of various sizes. The sun rotates. But curiously, the convection zone rotates differentially, with the equatorial regions rotating faster than the polar regions. So, a spot near the equator of the sun takes 25 days to complete a loop, whereas a spot near the pole takes about 33 days. Of course, the sun can “twist” this way because it is gas.
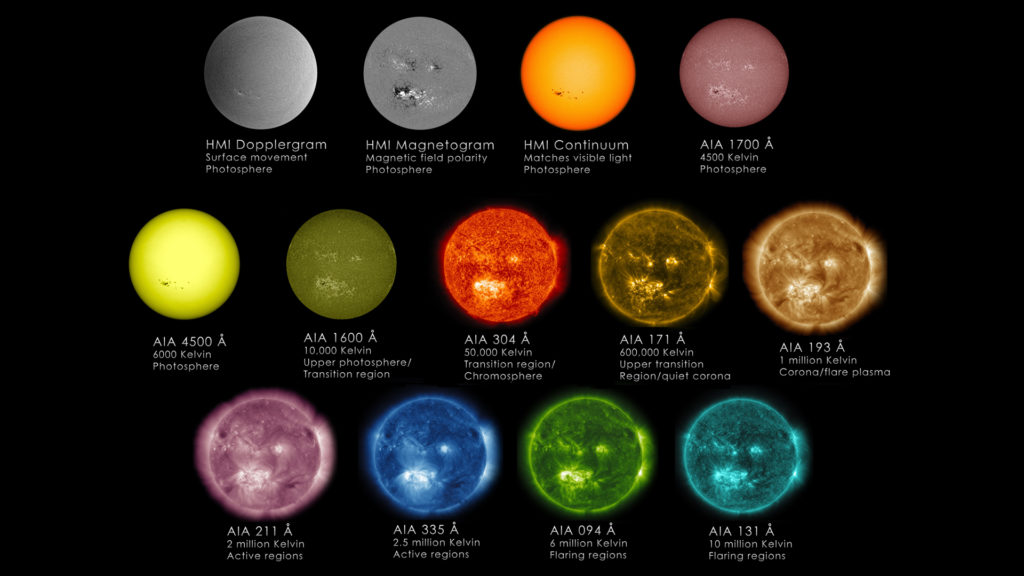
Below the convection zone is the radiative zone. The radiative zone is characterized by a lack of movement; it does not have the convection cells that are ubiquitous near the solar surface. And it exhibits solid-body rotation. This doesn’t mean it is solid, but rather that it rotates at the same rate everywhere, just as all solid objects must do. Below the radiative zone is the solar core. The temperature of the core is around 15 million degrees Celsius. This is so hot that the hydrogen nuclei experience nuclear fusion. So, hydrogen is constantly fusing into helium in the solar core; this releases energy which gradually trickles up to the surface.
But how can we possibly know what goes on below the photosphere? The answer is found in a branch of science called helioseismology. On Earth, we can learn about the interior of our planet by measuring the vibrations of Earthquakes at various locations. Earthquakes create waves that travel through the Earth’s interior, and these waves are bent in a specific way depending on the density and temperature of the material. This is how we know about the interior of the Earth. This branch of geophysics is called seismology.
Likewise, the sun experiences “sun quakes” – powerful vibrations along its surface. But unlike the Earth which experiences quakes only occasionally, the solar surface is always producing quakes everywhere. There are powerful mathematical techniques scientists use to reconstruct how the waves from such quakes have moved through the solar interior. This is how we know about the motion, densities, and temperatures of the solar interior.
There is another way we have direct information about the solar core. The process of nuclear fusion generates particles called neutrinos. They are similar to electrons except they are much less massive and have no electric charge. Being chargeless, they are not influenced by the charges of the electrons or protons in an atom. Hence, neutrinos can pass through ordinary matter. These ghostly particles are produced by fusion in the solar core, and pass straight through the outer layers of the sun at nearly the speed of light. We can detect these particles here on Earth, and have confirmed that they are indeed coming from the sun. This confirms that the sun is indeed fusing hydrogen in its core, and we know from quantum physics that this process requires a temperature of around 15 million degrees.
The Faint Young Sun Paradox
The same physics that tells us how nuclear fusion works also poses a challenge to those who reject the biblical timescale in favor of billions of years. Since the solar core is constantly fusing hydrogen into helium, the composition of the sun’s core should gradually change, decreasing in hydrogen and increasing in helium. In the biblical timescale of about 6000 years, the effect is negligible. However, the end result of this is that sun should gradually brighten over billions of years. Hence, if the sun were truly 4.5 billion years old, then it must have been much fainter in the past.
In fact, the sun would have been about 30% fainter in the distant past, when life was supposedly starting to evolve on Earth. But that’s a problem, because the Earth would have been completely frozen with so little solar energy. There is no evidence from geology that the Earth ever had such conditions. If anything, geology and paleontology suggest that the Earth was a bit warmer in the past. This is an inconsistency with the secular model. But the evidence is fully consistent with biblical creation.
Designed
The sun is designed to accommodate life on Earth. It is the right distance and right temperature for the Earth to have liquid water – an essential ingredient for life. Some stars are far hotter and brighter than the sun. In order for a planet orbiting a blue star to have the right temperature for life, it would have to orbit at a much greater distance. But blue stars emit far more ultraviolet radiation than the sun. Such radiation is destructive to life. Red dwarf stars are far cooler and fainter than the sun. To have liquid water, a planet orbiting a red dwarf would have to orbit much closer than Earth does. But red dwarf stars produce far less visible light in proportion to infrared radiation than the sun does. The sky of such a world would be considerably darker than that of Earth.
The sun unusually stable. Many stars pulsate, drastically changing their size and brightness over time, sometimes in a matter of days. Such pulsations would produce extreme temperature variations on any planet in orbit, making life impossible. Some stars experience superflares – powerful surface explosions caused by released magnetic field energy. Such explosions would be lethal if we were orbiting such a star. But the flares on the sun are mild, only about one ten thousandth the power of a superflare. The Lord designed the sun to do what it does; and it does it very well. More to come.