Thirty years ago, the only known planets were those in our solar system. But now, astronomers have detected over 4,800 planets orbiting other stars in our galaxy. These extrasolar planets challenge secular origins scenarios and confirm biblical creation.
Introduction
From ancient times, people have watched the sky and mapped the positions of the stars. These constellations appear the same from year to year, and century to century. But sky watchers of the past also noticed five unique stars that do not maintain a fixed position. These “wandering stars” move back and forth from one constellation to the next in a way that was difficult for ancient astronomers to predict. We refer to these oddities as planets from the Greek word meaning wanderer.
The planets known to the ancient world were Mercury, Venus, Mars, Jupiter, and Saturn. By the 1600s, many astronomers came to understand that Earth is also a planet, and that it orbits the sun along with the other five in an elliptical (almost circular) path. This understanding allowed astronomers to understand the motion of the planets, and to predict their positions with great accuracy.[1] The invention of the telescope in 1608 would allow us to eventually discover planets that are too faint to be seen with the unaided eye. This resulted in the discovery of the planet Uranus in 1781, of Neptune in 1846, and of Pluto in 1930. Of course, Pluto was much smaller than the other 8 planets, and was eventually reclassified as a dwarf planet in 2006.
The planets orbit the sun, which is why they shift from one constellation to the next over months or years. But the background stars are “suns” in their own right, and are at much greater distances. Astronomers had long suspected that many of these stars might have their own system of orbiting planets. These would be called extrasolar planets or exoplanets. However, the extreme distance to the stars made direct observations of such planets nearly impossible.
The Difficulty
Even a very modest backyard telescope will allow you to see the planets in our solar system, and even surface details on some of them. These planets shine by reflected sunlight.[2] And since we are in the same solar system, the angle between a planet and the sun as seen from Earth can be very high. Thus, we can view a planet after sunset, when the bright glare from the sun is blocked by the Earth, and yet the planet we are viewing is not. So, although the sun is a billion times brighter than Venus, we can easily see Venus when the sun is blocked by the Earth.
But this technique will not work for planets orbiting other stars. Viewing one solar system from another means that the planets will appear very close in angle to their star as seen from our location. The farther the star from us, the smaller the angle for a planet orbiting its star at a given distance. Consider our nearest neighbor: Proxima Centauri.[3] Proxima Centauri is 4.247 light years away, or 24.96 trillion miles. If an earthlike planet were orbiting Proxima at the same distance Earth orbits the sun, what would be the maximum angle between it and its star as seen from Earth? The answer is 0.77 arcseconds, or about the width of a penny as seen from a distance of three miles. Imagine trying to spot a point of light that is only 0.77 arcseconds away from another point of light that is a billion times brighter. That is the challenge of trying to detect extrasolar planets by direct observation.[4]
Pulsar Planets
Due to these difficulties, the first method by which exoplanets were detected was not by direct observation, but by an ingenious application of Newton’s laws. A star exerts a gravitational force on a nearby planet that bends the planet’s path into an orbit. The planet exerts an equal and opposite force on the star – that’s Newton’s third law. Therefore, a star and planet actually orbit each other around their common center of mass which is usually inside the star. Therefore, it is possible to detect a planet by measuring the slight wobble it gravitationally induces on its host star. The first three planets outside our solar system were discovered by this method. Curiously, these planets do nor orbit a sunlike star at all, but rather a pulsar.
A pulsar is a small, compact object only about the size of a city. However, it has a mass greater than that of the sun. Pulsars are sometimes produced when a massive star explodes in an event called a supernova.[5] The inner portions of the star are compressed by the explosion into a small sphere which forces the electrons in the atoms into the nucleus where they combine with protons to form neutrons. This city-sized sphere of pure neutrons is called a neutron star. Conservation of angular momentum causes this sphere to spin very rapidly. Powerful magnetic fields beam radiation away from the star. If one of these beams sweeps past the direction of our solar system, we detect a powerful radio pulse every time the star rotates. When a neutron star is aligned this way, we call it a pulsar.[6]
The radio signals from a pulsar are extremely regular due to the rotation of the star. Thus, any deviation in the timing must be due to the fact that the pulsar has moved a bit closer or farther from our location. Astronomers found that the pulsar PSR B1257+12 had slight variations indicating that it was wobbling. These wobbles were induced by the gravitational tug of three orbiting planets. The variation in the pulses allowed astronomers to deduce the exact periods and approximate masses of these three planets. Two of these planets were discovered in 1992, and the third was detected in 1994. They have been named Draugr, Poltergeist, and Phobetor. All three of these planets orbit closer to the pulsar than Earth orbits the sun. The innermost planet, Draugr is only 2% the mass of Earth, whereas the other two are both about four times the mass of Earth.
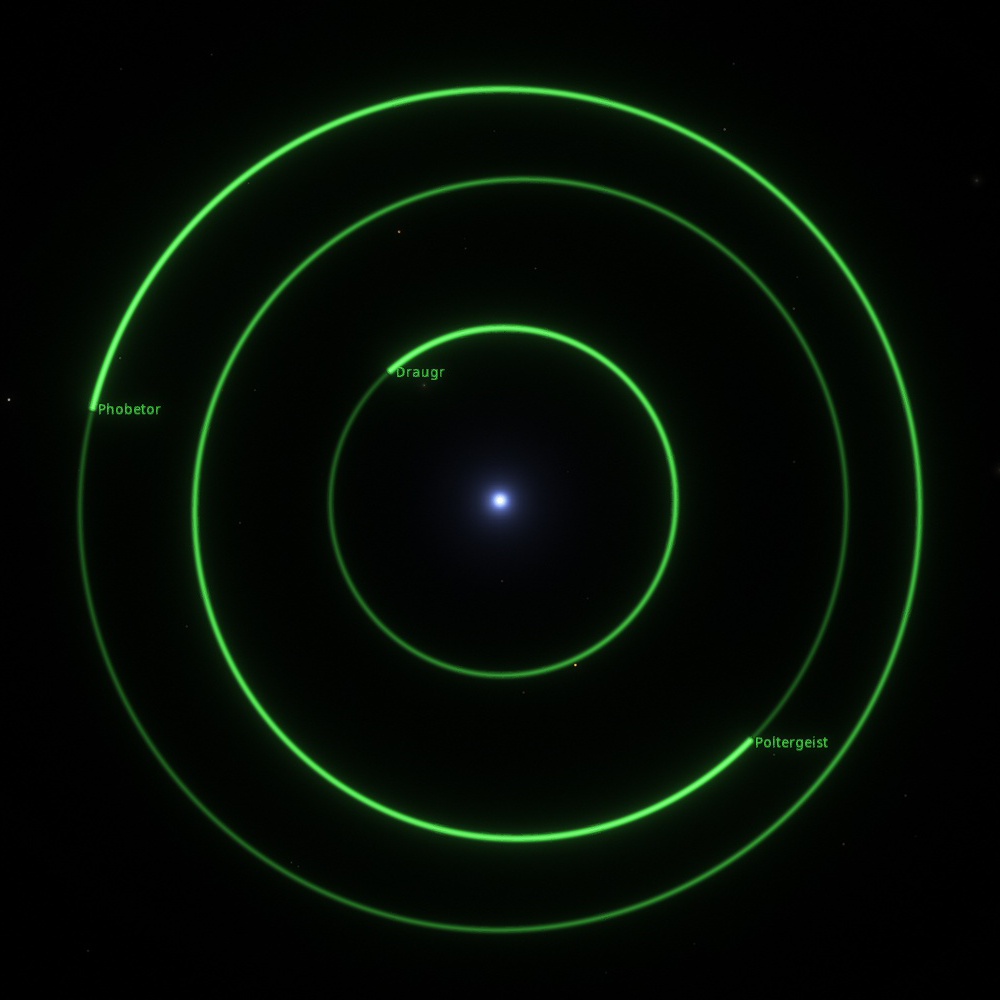
The discovery that pulsars can have planets is challenging to the standard, secular model of stellar evolution. When a star explodes, most of its mass is ejected into space. Therefore, any previously orbiting planets would be traveling in excess of escape velocity around the pulsar, and should fly off into space. However, from a creation perspective, it is possible that some pulsars are part of the original creation, and therefore may have been created with orbiting planets.
The Doppler Shift Method
“Regular” (main-sequence) stars do not emit precise radio pulses like pulsars. Nonetheless, there is a way to detect any wobble due to an orbiting planet: the Doppler shift. When we break the light of a star into a spectrum, we notice that certain frequencies of light are strongly attenuated. These absorption lines are due to the electron levels of the elements in the star – a sort of atomic fingerprint. This tells us the surface temperature and composition of the star. And it also tells us how the star is moving. If a star is moving toward an observer, its spectral lines will be shifted toward the blue end of the spectrum due to the Doppler effect. If moving away, the lines are redshifted. By measuring the tiny change in Doppler shift over time, we can measure the change in radial velocity of a star due to the gravitational influence of orbiting planets.
The first planet discovered orbiting a normal star was detected by this method in 1995. The star, 51 Pegasi, was found to wobble with a precise period of 4.23079 days. The planet must orbit at a distance of only 0.05 AU in order to have such a rapid period. (One AU is defined as the average distance between the Earth and the sun – about 93 million miles). Thus, the planet orbits 51 Pegasi seven times closer to its star than Mercury is from the sun!
Extrasolar planets are named after their star, followed by a lowercase letter indicating the order of their discovery, starting with “b.” So, 51 Pegasi b is the first planet to be discovered orbiting the star 51 Pegasi. The planet was also given the informal name Bellerophon. However, in 2015, the International Astronomical Union approved the official proper name for this planet as Dimidium.[7]
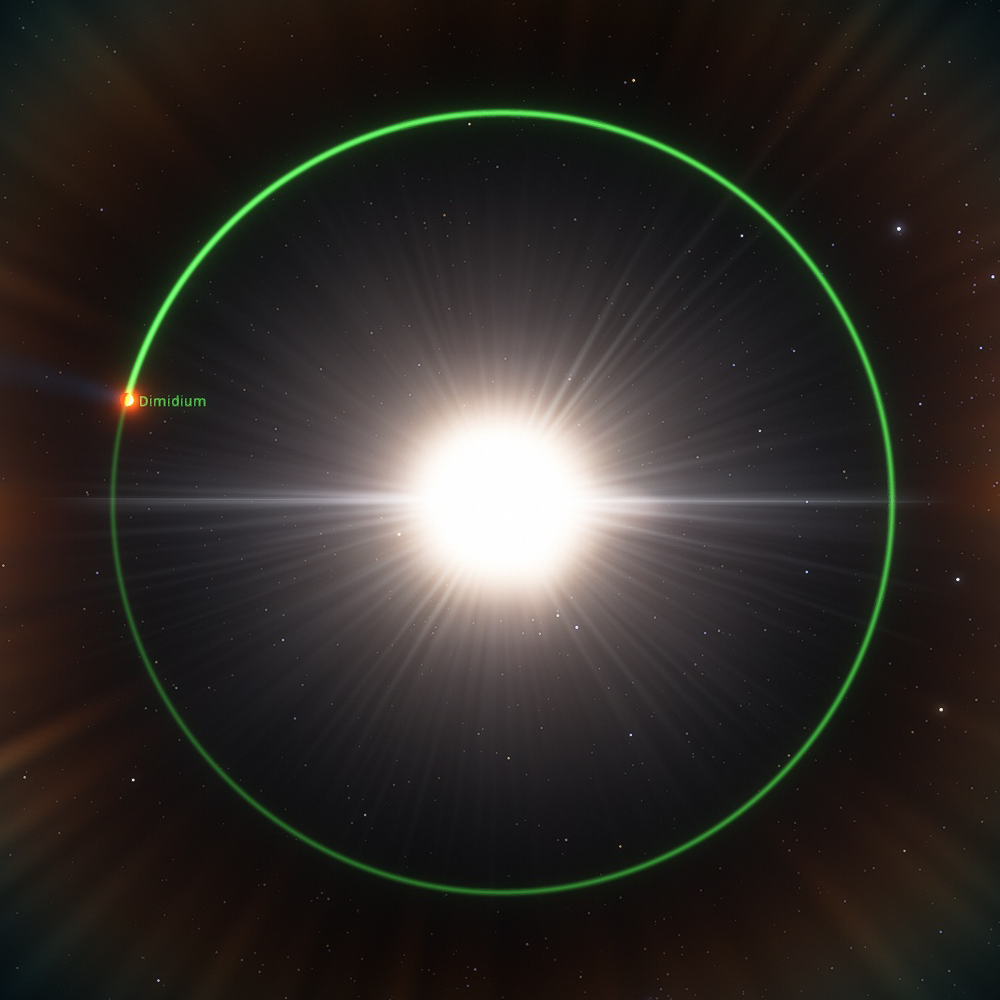
The amplitude of wobbling also allows us to estimate the minimum mass of the planet, in this case, about half the mass of Jupiter. If we knew the orientation of the system (anywhere from edge-on to face-on), we could compute the actual mass. In other words, if the system is nearly edge-on from our perspective, then the mass of Dimidium is indeed about half the mass of Jupiter. But if the system is tilted at a high angle, then the planet’s actual mass is much greater than that of Jupiter. Either way, it is clear that this is a gas giant planet, and yet it orbits very close to its star. It’s a “hot Jupiter.”
As additional planets were discovered by the Doppler method, many of them also turned out to be hot Jupiters. These are the easiest type of planet to detect by the Doppler method. Yet, the existence of hot Jupiters was contrary to the predictions of secular solar system formation models. This is because gas giant planets are supposed to form relatively far from their star, at distances comparable to the giant planets in our own solar system. The extremely strong stellar winds of young stars are supposed to strip away the hydrogen envelope of any planets that orbit close to the star, leaving only rocky, terrestrial planets like Mercury and Venus. Yet, since solar systems were created by God rather than forming naturally, there is no reason why we can’t have hot Jupiters from a creationist perspective.
Using the Doppler shift method, we now know that our nearest stellar neighbor, Proxima Centauri, does indeed have at least two planets. Proxima Centauri b is at least as massive as Earth, and orbits its star every 11.18 days at a distance of only 0.0485 AU; that’s nearly eight times closer to its star than Mercury is to the sun. Despite its closeness to the star, Proxima Centauri b likely has a temperature similar to, or perhaps slightly cooler than that of Earth. This is because Proxima Centauri is a red dwarf star, cooler and far less luminous than the sun. Proxima Centauri c orbits at a distance of 1.49 AU (comparable to Mars) with a period of 5.3 years. The planet is about seven times the mass of Earth.
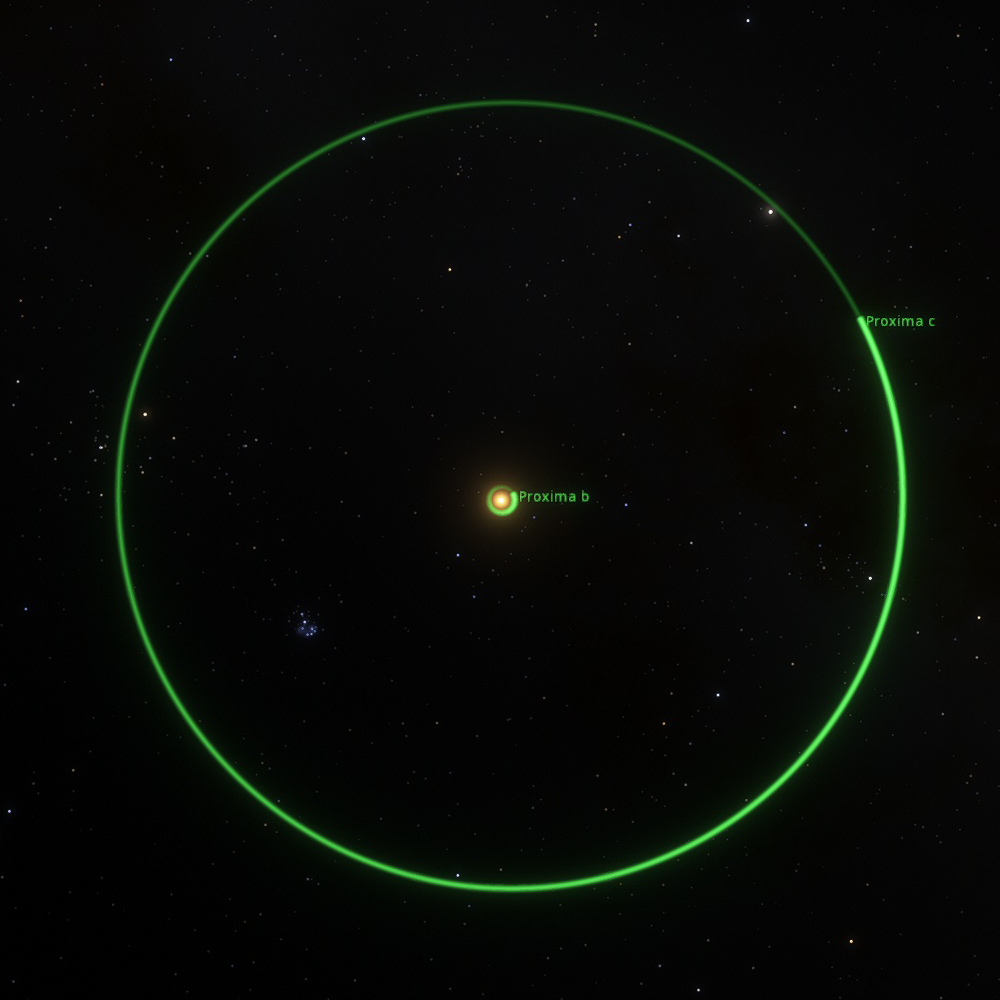
Direct Observations of Exoplanets
Amazingly, despite the many challenges involved, a few extrasolar planets have been directly imaged. This is achieved either by physically blocking the star and allowing its immediate surroundings to be imaged, or by using mathematical techniques to reduce the glare from the star to reveal planets. Clearly, this technique is only possible for relatively nearby stars whose planets orbit at a great distance from their star. The first planet to be confirmed by this method was Fomalhaut b. Hubble Space Telescope images from 2004, 2006, 2010, and 2012 show this planet slowly orbiting its star, just inside a ring of orbiting material. This planet has also been given the name Dagon, after the god of the Philistines whose statue fell down before the Ark of the Covenant (1 Samuel 5:1-5). Fomalhaut is one of the brighter stars in our night sky. It is found low in the south in Autumn evenings for northern hemisphere viewers.
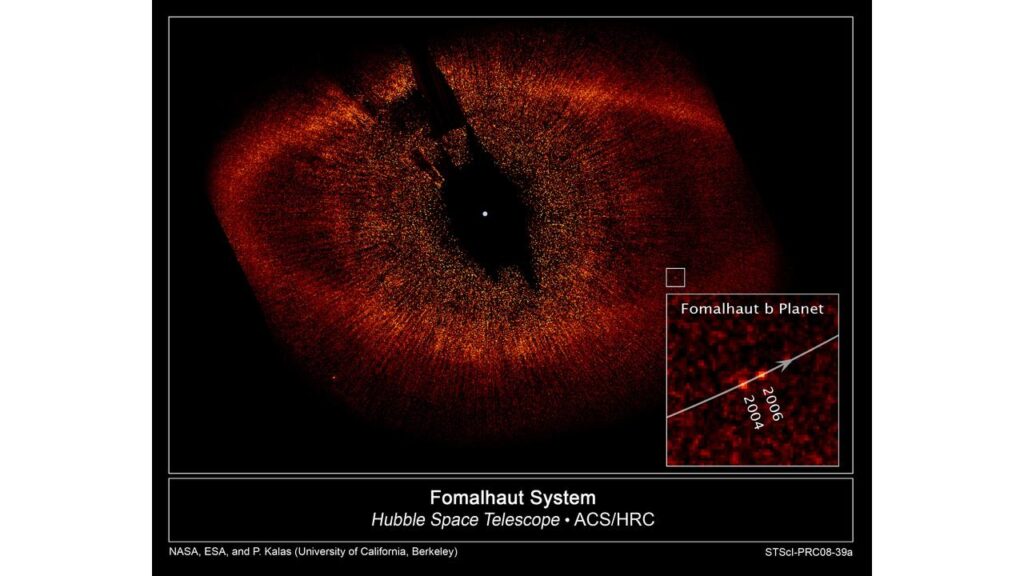
Further studies of Dagon have been perplexing. There is a curious lack of infrared radiation normally associated with giant planets. This suggests that the light from Dagon may be primarily reflected light from Fomalhaut off of a cloud of dust or a massive ring system surrounding the planet – much more extensive than Saturn’s rings. Some astronomers have suggested that Dagon may not actually be a planet, but a dust cloud from a recent collision of asteroids. However, such a cloud would disperse in a decade or so. In light of the extraordinary timing, this seems an improbable explanation. The upcoming James Webb Space Telescope may help resolve this controversy.
Another star whose planets have been directly imaged is HR 8799. This discovery was announced in 2008 at the same time as the discovery of Fomalhaut b. Amazingly, astronomers have directly observed four planets orbiting this star. Images taken with the Keck telescope over a span of seven years clearly show the motion of these planets, as shown in the time-lapse video. We are so blessed to live at a time where we can actually see planets orbiting other stars!
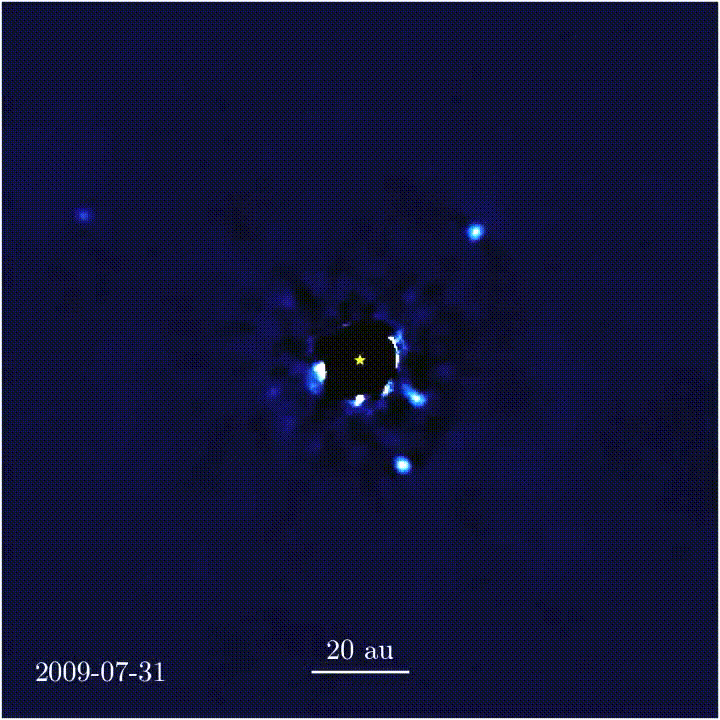
The innermost planet, HR 8799 e, is estimated to have an orbital period of about 40 years. It orbits at 15 AU. If it were in our solar system, that would place it somewhere between the orbits of Saturn and Uranus. The outermost planet, HR 8799 b, is estimated to have an orbital period of over 400 years at a distance of about 70 AU (over twice the distance between Neptune and the Sun). The exact periods are unknown, partly because we have not yet observed a complete orbit for any of these worlds, and partly because we do not know the precise orientation of each orbital plane. Some astronomers suspect that the four planets may be in a near 1:2:4:8 orbital resonance, where the period of each planet is twice that of the one immediately interior.[8] Such a configuration would be extremely stable; in fact, three of Jupiter’s moons are in such a resonance.[9]
All four planets orbiting HR 8799 are gas giants, like Jupiter, but larger. If there are also smaller, rocky planets orbiting much closer to the star (as is the case in our solar system), they are too faint to be detected by current technology owing to the glare of the star itself. This stellar system is 133 light years away from ours. Although the star is about 5 times the luminosity of the sun, from our solar system it appears as a very faint star, near the threshold of what we can see with the unaided eye under ideal conditions. The star is easily visible in binoculars and is found in the constellation Pegasus.
Another noteworthy stellar system is that of the star PDS 70. This star is orbited by a thick disk of dust at a distance of 140 AU, and by two planets, all of which have been directly imaged. The inner planet, PDS 70 b, orbits at 22.7 AU, and is about the same mass as Jupiter. The outer planet orbits at 30.2 AU and is 4 times the mass of Jupiter. As of 2021, PDS 70 and HR 8799 are the only star systems with multiple planets that have been directly imaged. Nearly 50 other extrasolar planets have also been directly imaged, but in each case only one planet is observed per star.
More to Come
However, the method that has resulted in the majority of the known 4800 exoplanets is neither the Doppler method, nor direct imaging. It is instead the transit method. Although this method works on only a small fraction of stellar systems, there are apparently so many such systems that the transit method dominates. We will explore this method in the next entry.
[1] The key was to recognize that we are on a moving planet watching other moving planets. Once this was understood, the behavior of the planets was straightforward.
[2] We know that planets shine by reflected sunlight because they experience phases like the moon. For example, when the sun is to the right side of a planet, only the right side is illuminated.
[3] Proxima Centauri is the smallest star of the Alpha Centauri star system. The Alpha Centauri system contains three stars, named A, B, and C (Proxima) in order of decreasing size, mass, and brightness. A and B orbit each other every 80 years and are separated by an average distance of 2.2 billion miles. Proxima orbits at a much greater distance of about 1.2 trillion miles.
[4] Atmospheric turbulence normally causes blurring of telescopic images in excess of 1 arcsecond. However, under ideal circumstances, it is possible to get down to 0.1 arcsecond of clarity with a powerful telescope. And so you might think that a 0.77 arcsecond separation would be easy to spot. However, the star would be around 1 billion times brighter than the planet. Glare is the issue. For comparison, many amateur astronomers enjoy the challenge of spotting Sirius B – a white dwarf that orbits the star Sirius A. Depending on the year, the separation between these two is between 3 and 11 arcseconds. But Sirius A is about 9000 times brighter than Sirius B, which makes spotting the white dwarf quite a challenge. Now consider reducing the distance between the two by a factor of ~10, and increasing the brightness difference from 9000 to 1 billion, and you will understand the challenge of direct imaging of extrasolar planets.
[5] The pulsar in the center of the Crab Nebula (M1) apparently resulted from a supernova that occurred in A.D. 1054. This supernova was recorded by eye-witnesses, and produced the (still expanding) Crab Nebula.
[6] So, all pulsars are neutron stars, but not all neutron stars are pulsars. Only those aligned such that we receive the radio pulses are pulsars. Thus, a neutron star could be a pulsar as viewed from one solar system, and yet not a pulsar as viewed from a different solar system.
[7] https://www.iau.org/news/pressreleases/detail/iau1514/
[8] Wang, Jason J., et al., Dynamical Constraints on the HR 8799 planets with GPI, The Astronomical Journal, 2018, Volume 156, Number 5, p. 192
[9] Io, Europa, and Ganymede are in a 1:2:4 orbital resonance.